End-of-Life: a neglected pillar of Life-Cycle Assessment
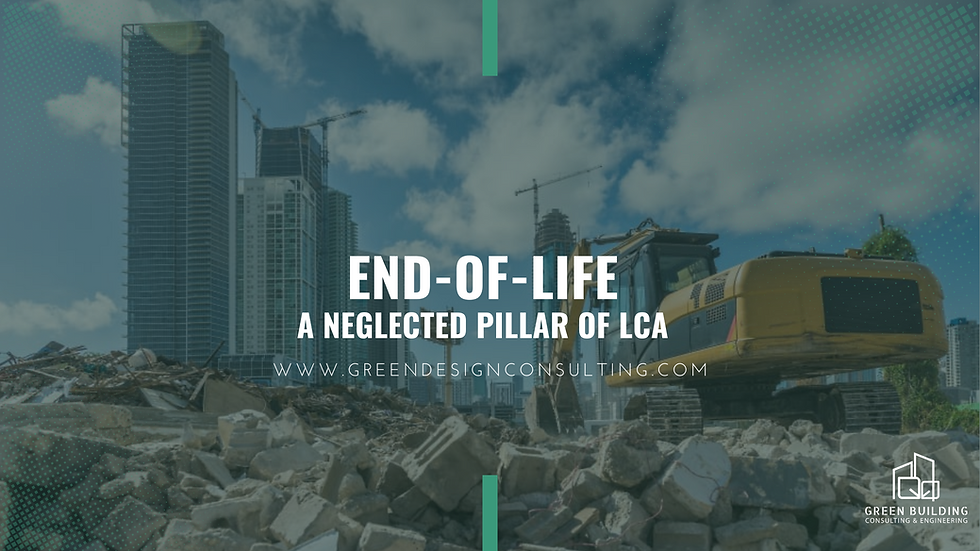
The Life Cycle Assessment (LCA) is now an essential tool for evaluating the environmental impact of buildings. By incorporating every phase, from material production to end-of-life management, the LCA allows for measuring and optimizing the sustainability of projects.
The EN 15978 standard defines the key stages of a building’s life cycle, but phases C (end-of-life) and D (reuse) are often overlooked, despite being critical. Deconstruction, waste management, and material reuse have a significant impact on the overall environmental balance, particularly in terms of reducing CO₂ emissions and minimizing consumed resources. These phases are also the ones that close the loop, ensuring circularity in buildings. Therefore, it is crucial to include them in any sustainability strategy.
LCA and its Phases
The Life Cycle Assessment (LCA) quantifies the environmental impacts of buildings over their entire lifespan. Structured by the EN 15978 standard, it is divided into several essential phases: production, construction, use, and end-of-life, with a potential for reuse. Each phase presents its own challenges and opportunities for sustainability.
The EN 15978 standard is widely used in Europe to assess the environmental performance of buildings throughout their life cycle. While this standard is primarily applicable in European countries, it also influences sustainable construction policies through certifications like LEED and BREEAM, which draw on its principles to standardize the assessment of buildings in terms of ecological impact. The standard is also recognized in other regions, including the UK and some parts of Asia, though it remains closely tied to European initiatives.
Summary of the Phases
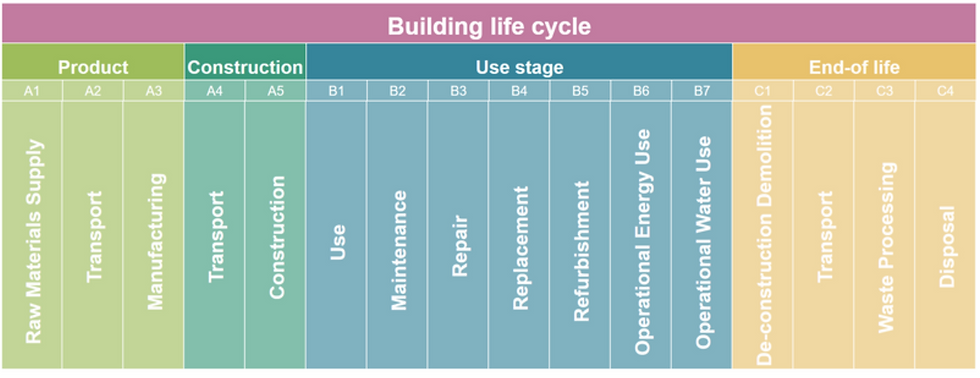
Phase A: Production (A1-A3)
The production phase involves the extraction of raw materials, their transportation, and the manufacturing of construction products. Material selection is crucial here—concrete, for instance, is energy-intensive, while wood may have a lower environmental impact. This phase accounts for both direct emissions from industrial processes and transport-related emissions. Optimizing materials and sourcing can significantly reduce the ecological footprint from the early stages of a building's life cycle.
Phase A: Construction (A4-A5)
This phase covers the transportation of materials to the construction site and the building activities themselves. Transport is a significant source of CO₂ emissions, especially if materials are sourced from far away. On-site processes, such as foundation preparation and structure assembly, consume energy and generate waste. Reducing transport distances and using efficient construction techniques are key strategies for minimizing environmental impacts.
Phase B: Usage (B1-B7)
The use phase is the longest in the building's life cycle, encompassing energy and water consumption, as well as maintenance, repair, and replacement operations. During this phase, the energy performance of the building becomes particularly important. Design choices, such as insulation and energy-efficient systems, directly impact long-term consumption. The durability of construction materials also influences the frequency and intensity of maintenance needs.
Phases C et D: Fin de vie et réutilisation
Phases C and D focus on the building's end of life and the potential for material reuse. Phase C involves the deconstruction or demolition of the building and the management of the resulting waste, which can be recycled, repurposed, or sent to landfill. Phase D targets the reuse of materials for new projects, reducing the need to extract new resources.
Phase C – End-of-Life
Phase C, focused on the end-of-life stage of buildings, includes deconstruction or demolition, as well as waste management. This process is a crucial component of Life Cycle Assessment (LCA) as it directly contributes to reducing greenhouse gas emissions associated with the disposal of materials. At this stage, the amount of waste that can be recycled or reused is critical in limiting the overall environmental impact of a project. However, the end-of-life phase is often underestimated in environmental assessments, despite its role in determining the circularity of materials. The importance of this phase lies not only in minimizing landfill waste but also in optimizing the recycling potential of materials.
In Singapore, the Building and Construction Authority (BCA) has implemented LCA requirements for future building developments, emphasizing the importance of this phase. Recent policies in Singapore mandate the integration of LCA in the design and construction process, with a strong focus on reducing carbon emissions through efficient material use and recycling strategies at the end of a building's life. This has been demonstrated through selective demolition practices, which have maximized the recovery and recycling of construction materials in several projects, significantly reducing CO₂ emissions. [https://www1.bca.gov.sg/buildsg/sustainability/regulatory-requirements-for-existing-buildings]
The challenges posed by Phase C are multifaceted. First, the logistical infrastructure for collecting and processing construction waste is often inadequate in certain regions, particularly in Southeast Asia, where recycling options are limited due to a lack of specialized facilities. Second, the costs associated with sustainable waste management can be prohibitive, discouraging many projects from adopting green practices. Finally, the absence of unified standards in many countries makes the implementation of consistent solutions difficult, exacerbating the complexity of material management at the end of life.
Phase D – Reuse and Recovery
Phase D, focused on the reuse and recovery of materials from deconstruction, is a critical lever for integrating circular economy principles into the building sector. When a building reaches the end of its life cycle, managing the recovered materials can drastically reduce the consumption of new resources. Rather than sending these materials to landfills, they can be reused in new projects, which not only minimizes environmental impact but also cuts down on the costs associated with the extraction of raw materials.
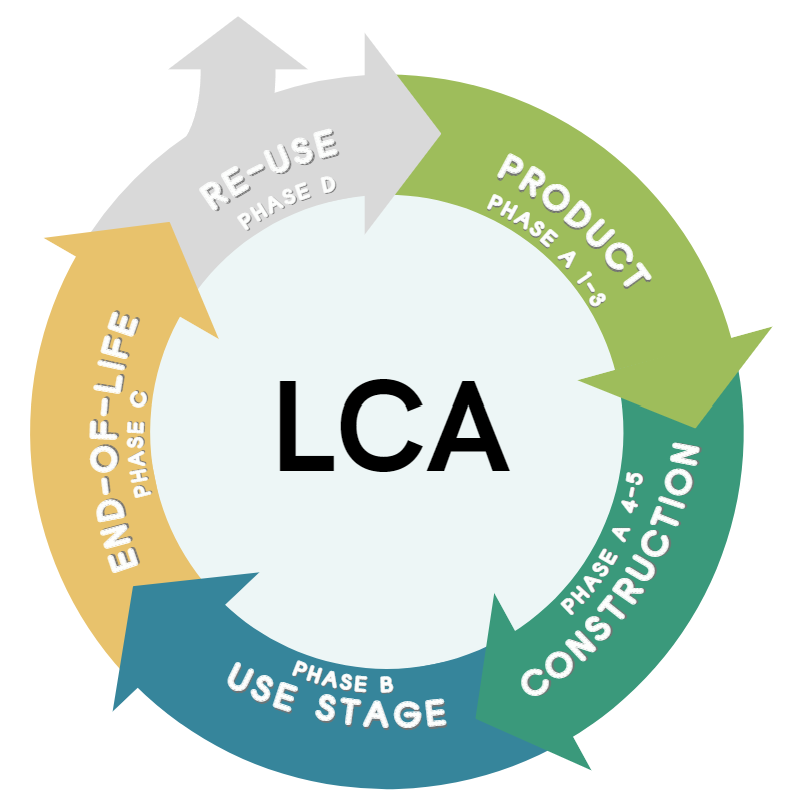
A remarkable example of material reuse in a deconstruction project can be found in the deconstruction of the Tokyo Olympic venues in Japan. After the 2020 Olympics, large parts of the temporary structures were dismantled, and the materials, including steel and concrete, were reused in new urban developments. This approach significantly reduced the carbon footprint of the new construction phase, showing how intelligent management of demolition materials can minimize environmental impact while contributing to the preservation of resources [https://olympics.com/ioc/news/tokyo-2020-s-olympic-venues-combine-tradition-with-the-future]
The environmental benefits of this phase are undeniable. On one hand, the reuse of materials reduces the strain on natural resources and ecosystems linked to extractive industries. On the other hand, it significantly decreases the amount of waste directed to landfills, contributing to a reduction in greenhouse gas emissions, especially methane. However, this phase is not without challenges. The technical mastery of selective deconstruction, high initial costs, and the lack of specific expertise in some regions can hinder the widespread adoption of these practices. Although the need for expertise in this phase is increasingly recognized in higher education, it remains rare.
Conclusion
Phases C and D of a building’s life cycle, often underestimated, play a fundamental role in assessing overall environmental impact. The end-of-life treatment of materials and their reuse are crucial levers for optimizing project sustainability. By valorizing materials from deconstruction, as demonstrated in the Tokyo Olympic venues project, CO₂ emissions can be reduced and resource consumption minimized.
It is imperative to systematically integrate these phases into the sustainability strategies of construction projects. Industry stakeholders must implement solutions aimed at maximizing material reuse and recycling while considering the associated economic and technical constraints. Emerging technologies such as BIM (Building Information Modeling) and AI offer promising perspectives for optimizing the management of material life cycles. These tools enable not only more precise planning of deconstruction operations but also better anticipation of material reuse potential.
Interested in learning more about our services? Contact us!
Written by Mehdi BELAHOUCINE
Comments