Carbon-Negative Building Materials: A Sustainable Revolution
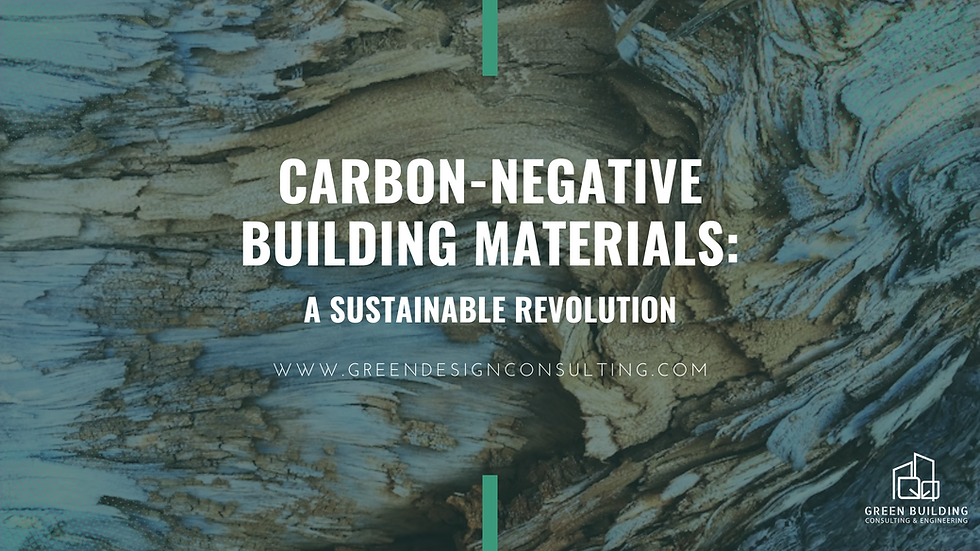
In the current fight against climate change, the building industry plays a key role in reducing greenhouse gas emissions. Did you know that the construction sector is responsible for nearly 39% of global CO₂ emissions [1]? This includes direct emissions from the materials used, as well as those related to production and transport. Faced with this challenge, the use of carbon-negative materials emerges as a solution for the future [2]. These materials do not just limit their environmental impact—they actively capture and store carbon throughout their lifecycle.
In this article, we will explore the benefits of these materials and innovative examples of their application in the building sector.
What is a Carbon-Negative Material?
Definition and Explanation of the Concept
A carbon-negative material is one that, over its entire lifecycle, captures more carbon dioxide (CO₂) than it emits [3]. This process includes raw material extraction, production, installation, usage, and even the material's end-of-life phase. Unlike traditional materials that release significant amounts of CO₂ into the atmosphere, carbon-negative materials actively help reduce greenhouse gas emissions.
These materials capture carbon through various natural or technological processes. For example, bio-based materials like wood or hemp naturally absorb CO₂ from the atmosphere during their growth, while others, like specific concretes, integrate carbon capture technologies during their production.
Difference with Carbon-Neutral Materials
Carbon-neutral materials, while eco-friendly, differ from carbon-negative materials. Carbon-neutral materials emit as much CO₂ as they capture or offset throughout their lifecycle, resulting in a net-zero emissions balance [4]. In contrast, carbon-negative materials go further: they actively remove CO₂ from the atmosphere, surpassing mere emissions neutrality and proactively reducing the overall concentration of carbon in the air.
Benefits for the Construction Sector
The use of carbon-negative materials in construction offers significant environmental benefits. By reducing the amount of CO₂ in the atmosphere, these materials contribute to the fight against climate change while making buildings more sustainable. Their adoption also:
Enhances environmental certifications such as LEED or BREEAM, which recognize low-carbon buildings.
Reduces dependence on polluting materials by promoting the use of renewable and low-impact resources.
Optimizes building energy performance, as some materials provide insulating or temperature-regulating properties, thereby lowering energy needs.
By integrating these materials, the construction sector can play a key role in transitioning to more sustainable practices while meeting growing environmental regulatory requirements.
Types of Carbon-Negative Materials
Bio-Based Wood
Wood is one of the most promising materials for sustainable construction, particularly due to its ability to store CO₂. As a tree grows, it absorbs carbon dioxide through photosynthesis, storing it in its fibers. This CO₂ remains trapped throughout the tree’s life and even after it is harvested, as long as the wood is not burned or decomposed. One cubic meter of wood can store approximately 1 ton of CO₂ [5], making it an excellent material for reducing carbon emissions in the construction sector.
Laminated timber structures are increasingly common in architectural projects. This type of wood, made from multiple layers glued together, is not only strong [6], but it also allows for the construction of taller, more complex buildings. For instance, iconic projects like the Mjøstårnet in Norway [7], the tallest wooden tower in the world, use laminated wood, showcasing the potential of this material in modern low-carbon constructions.
Finally, for wood to truly be a carbon-negative material, sustainable forest management is essential. This involves planting new trees to replace those harvested, preventing illegal deforestation, and using certifications like FSC (Forest Stewardship Council) [8] to ensure the sustainability of forest resources.
Carbon-Capturing Concrete
Although concrete traditionally has a high carbon footprint, innovations are transforming it into a carbon-negative material. Concrete can capture CO₂ during its curing phase through a process called carbonation [9]. During this process, CO₂ reacts with calcium hydroxide in the concrete to form calcium carbonate, which permanently locks the carbon dioxide into the material. This mechanism helps reduce the net emissions associated with concrete production.
Pilot projects have already demonstrated the effectiveness of this innovative concrete. For example, the startup CarbonCure has developed technology that injects captured CO₂ into concrete during manufacturing, thereby reducing the carbon footprint of construction. Infrastructures such as parking structures and industrial buildings are already using this type of concrete, showing promising results in terms of CO₂ emission reductions [10].
The widespread adoption of CO₂-absorbing concrete could mark a significant advancement in the construction sector, reducing the carbon impact of one of the most widely used materials in the world.
Bio-Based Insulating Materials: Hemp and Flax
Bio-based insulating materials like hemp and flax are increasingly praised for their ability to capture CO₂ and enhance building energy efficiency. Hemp, for example, is a fast-growing plant that absorbs significant amounts of CO₂ during its growth. When transformed into a building material, especially for thermal insulation, it continues to store this carbon throughout its life cycle.
The use of hemp in insulation and wall construction is becoming a viable option for eco-friendly construction. Hempcrete [11], a mixture of lime and hemp, is not only lightweight and insulating, but it also helps regulate humidity while capturing CO₂ during its production. This material is being used in innovative projects such as eco-homes in the UK and France, demonstrating its effectiveness both in reducing emissions and in providing thermal performance.
Flax is another plant with insulating properties and low environmental impact. Primarily cultivated in Europe, it can be transformed into insulating fibers for buildings. These fibers are not only renewable but also provide excellent thermal and acoustic insulation while having a low carbon emission footprint.
Projects incorporating these materials are multiplying, especially in residential buildings and renovation projects. In France, hemp and flax are increasingly used in passive houses and high-performance energy projects, offering a sustainable solution for future construction.
Advantages of Carbon-Negative Materials
Reduction of CO₂ Emissions
Carbon-negative materials play a key role in directly reducing CO₂ emissions. Due to their ability to capture and store carbon dioxide throughout their lifecycle, these materials help offset a portion of the emissions generated during building construction. By incorporating these materials into construction projects, the sector actively contributes to global climate goals, particularly those set by the Paris Agreement [12]. With the building sector responsible for nearly 40% of global CO₂ emissions [1], adopting carbon-negative materials becomes a tangible solution to mitigate the environmental impact of construction and facilitate the transition to a low-carbon economy.
Technical Performance and Durability
Beyond their contribution to reducing CO₂ emissions, carbon-negative materials offer superior technical performance. For example, bio-based materials like wood, hemp, or flax provide excellent thermal insulation, thereby reducing energy demand for heating and cooling in buildings [13]. Additionally, their lightness and strength make them easy to handle and integrate into construction projects, while also improving the building's energy efficiency.
These materials also have increased longevity, which helps reduce maintenance and upkeep costs. Properly treated wood from sustainably managed forests, for instance, can last for decades without losing its structural properties. Carbon-capturing materials like concrete are highly resistant to weather conditions and natural degradation, making them a durable and long-lasting solution for construction.
Environmental Certification and Compliance
Using carbon-negative materials facilitates the achievement of environmental certifications such as LEED (Leadership in Energy and Environmental Design) or BREEAM (Building Research Establishment Environmental Assessment Method). These certifications emphasize the integration of sustainable and low-impact materials in construction projects, enabling companies to meet the demands of environmentally-conscious clients while increasing the value of certified properties.
Furthermore, the adoption of these materials is encouraged by international regulations and standards. In a context where governments are imposing increasingly strict limits on CO₂ emissions and energy efficiency in buildings, the use of carbon-negative materials allows companies to comply with standards while anticipating regulatory developments. These materials thus become valuable assets for businesses aiming to lead in sustainable construction and adapt to evolving market expectations.
Challenges and Future Outlook
Current Limitations to Large-Scale Adoption
The widespread adoption of carbon-negative materials faces several barriers that hinder their large-scale deployment. One of the main challenges is the high initial cost. Compared to conventional construction materials, low-carbon or carbon-negative materials often require innovative production technologies, specific bio-based resources, or complex carbon capture and storage processes. As a result, these materials tend to be more expensive to produce, which translates into higher construction costs. For developers and investors, this financial hurdle can make adoption difficult, especially in projects with limited budgets.
Another significant challenge lies in the lack of knowledge and expertise within the construction sector. Most building professionals—whether architects, engineers, or contractors—are not yet trained in using these materials. Construction techniques involving cross-laminated timber or carbon-capture concrete, for instance, require specific skills and a thorough understanding of the unique benefits and challenges these materials present. Furthermore, the lack of concrete data on the long-term performance of some of these materials adds to the hesitation, as investors often prefer proven solutions.
Solutions to Accelerate Adoption
To overcome these obstacles, several solutions must be considered to promote the wider use of carbon-negative materials. First, ongoing technological innovations and research play a crucial role in reducing the production costs of these materials. Advances in carbon capture, bio-based material processing, and optimization of manufacturing processes are making these solutions more financially accessible. For example, pilot projects demonstrating the economic viability of using carbon-capture concrete in large-scale constructions are paving the way for broader adoption.
In parallel, public policy can stimulate the adoption of these materials. Subsidies and tax incentives for projects using low-carbon materials are essential to make these innovations more competitive in the market. Governments worldwide are beginning to implement regulations aimed at promoting sustainable construction, such as carbon emission reduction quotas for new buildings or environmental certifications favoring the use of carbon-negative materials.Education and training are also key to addressing the expertise gap in the sector. By incorporating modules on sustainable materials into the curricula for architects and engineers, and by offering ongoing training for building professionals, best practices can be more widely disseminated, boosting confidence in these solutions.
These combined actions—innovation, financial incentives, and professional skill development—are the levers that will help overcome current barriers and make carbon-negative materials a standard in the construction industry.
Conclusion
Carbon-negative materials represent a significant breakthrough in the pursuit of sustainable and environmentally friendly construction. By absorbing more carbon than they emit, these materials directly contribute to reducing CO₂ emissions, a critical challenge in the face of the climate emergency. Their widespread adoption has the potential to transform the construction sector, traditionally one of the most polluting industries, into a key player in the ecological transition. Additionally, bio-based materials such as wood, carbon-capturing concrete, and hemp and flax insulation provide high technical performance while reducing long-term maintenance costs.
However, for these materials to become standard in the construction industry, they must be supported by policies, economic incentives, and professional training. Through widespread adoption, carbon-negative materials can help meet global climate goals while creating more resilient buildings that can withstand environmental and economic challenges.
Finally, incorporating carbon-negative materials also facilitates the attainment of environmental certifications such as LEED or BREEAM, which have become essential for projects aiming for ecological excellence. At Green Building Consulting & Engineering, we guide companies and developers through the entire process—from design to certification—ensuring that each project reaches its full potential in terms of sustainability.
The adoption of these materials is not only an opportunity for the planet but also for the entire construction sector, which now has the chance to rethink its methods and become a driver of sustainable innovation.
[1] United Nations Environment Programme (2021). Global Status Report for Buildings and Construction 2021. https://www.unep.org/resources/report/2021-global-status-report-buildings-and-construction
[2] Churkina, G., Organschi, A., Reyer, C. P. O., Ruff, A., Vinke, K., Liu, Z., ... & Creutzig, F. (2020). Buildings as a global carbon sink. Nature Sustainability, 3(4), 269-276. https://www.congress.gov/116/meeting/house/110542/documents/HHRG-116-II00-20200226-SD004.pdf
[3] Levasseur, A., Lesage, P., Margni, M., Deschênes, L., & Samson, R. (2010). Considering time in LCA: dynamic LCA and its application to global warming impact assessments. Environmental science & technology, 44(8), 3169-3174. DOI: 10.1021/es9030003
[4] Nicholas Lippiatt, Tung-Chai Ling, Shu-Yuan Pan, Towards carbon-neutral construction materials: Carbonation of cement-based materials and the future perspective, Journal of Building Engineering, Volume 28, 2020, 101062, ISSN 2352-7102, https://doi.org/10.1016/j.jobe.2019.101062.
[5] Sohngen, B., & Mendelsohn, R. (1998). Valuing the impact of large-scale ecological change in a market: the effect of climate change on US timber. American Economic Review, 88(4), 686-710. https://www.researchgate.net/publication/4901430_Valuing_the_Impact_of_Large-Scale_Ecological_Change_in_a_Market_The_Effect_of_Climate_Change_on_US_Timber
[6] Hongbo Liu, Jianxiong Zhao, Jingxian Zhao, Zhihua Chen, Shixing Zhao, Shuheng Yang, Experimental study on the mechanical behavior of glulam suspendome considering the influence of prestressing, Journal of Building Engineering, Volume 95, 2024, 110180, ISSN 2352-7102, https://doi.org/10.1016/j.jobe.2024.110180.
[7] Saule Tulebekova, Kjell Arne Malo, Anders Rønnquist, Petter Nåvik, Modeling stiffness of connections and non-structural elements for dynamic response of taller glulam timber frame buildings, Engineering Structures, Volume 261, 2022, 114209, ISSN 0141-0296, https://doi.org/10.1016/j.engstruct.2022.114209.
[8] Forest Stewardship Council. (n.d.). Certification. https://us.fsc.org/en-us/certification
[9] Andrew, R. M. (2019). Global CO2 emissions from cement production. Earth System Science Data, 11(4), 1675-1710. https://doi.org/10.5194/essd-11-1675-2019
[10] CarbonCure Technologies. (2024). Studio Eugenia: CarbonCure concrete technology in action. https://www.carboncure.com/wp-content/uploads/2024/05/OP-StudioEugenia-1.pdf
[11] Kenneth Ip, Andrew Miller, Life cycle greenhouse gas emissions of hemp–lime wall constructions in the UK, Resources, Conservation and Recycling, Volume 69, 2012, Pages 1-9, ISSN 0921-3449, https://doi.org/10.1016/j.resconrec.2012.09.001.
[12] Höhne, N., Ellermann, C., & Fekete, H. (2014). The Paris Agreement: a global framework for climate action. German Institute for International and Security Affairs. https://www.idos-research.de/uploads/media/DP_34.2014.pdf
[13] Kharissova, Alena & Kharissova, Oxana & Kharisov, Boris & Peña, Yolanda. (2024). Carbon negative footprint materials: A review. Nano-Structures & Nano-Objects. 37. 101100. 10.1016/j.nanoso.2024.101100.
Comments